Space Exploration
What can we find in space? Is there any organisation to what we find? How do we discover what there is? These are all questions that can be answered to some degree by astrophysicists.
Objects in Space
Object | Description |
---|---|
planet | Object orbiting a sun with sufficient mass to form a spherical shape |
dwarf planet | A planet that is too small to be spehrical orbiting a sun, it may also have a very elliptical orbit |
moon | A natural object that orbits a planet |
Sun | The star around which we orbit, which contains 98% of the mass of our solar system. Our sun is also called Sol |
asteroid | a small rocky body orbiting the sun |
solar system | The Sun and all its satellite planets, their moons and the asteroid belt |
star | An object in space that is massive enough to undergo nuclear fusion, producing and emitting electromagnetic radiation |
exoplanet | A planet that has been discovered to exist outside our own solar system. One of the major techniques used to discover exoplanets is the tiny dip in the brightness of a star as a planet passes between the star and our telescopes. So far over 3000 exoplanets have been discovered (Jan 2023). |
galaxy | a system of millions or billions of stars, together with gas and dust, held together by gravitational attraction |
universe | All existing matter and space considered as a whole |
Along with all of these natural objects in space we have added satellites, space probes and a space telescope. There are many types of satellites, but only two principal heights of orbit.
Low Earth Orbit
Satellites in a low earth orbit are approximately 160 km to 2000 km above the surface of the planet. The International Space Station (ISS) is just over 400 km above the Earth's surface. It takes 90 minutes for the ISS to orbit the Earth once. The path that it takes passes over the north and south poles. All low earth orbits are either polar orbits or low-incilination orbits.
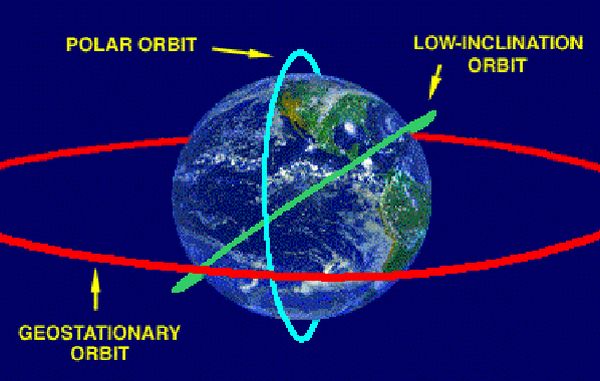
The image above is not to scale
These low orbits allow satellites to orbit the earth in about 90 minutes. This is very good for monitoring weather, global positioning system (gps), communications, environmental monitoring and scientific research. The Hubble Space Telescope orbits at a height of almost 600 km above the surface of the Earth.
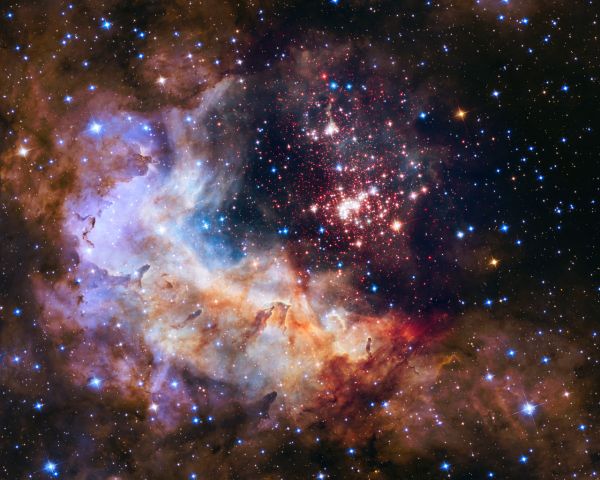
Westerlund 2 - Hubble's 25th Anniversary image
Geostationary Orbits
Satellites orbiting over the equator at a height of approximately 36,000 km above the surface of the Earth take 24 hours to orbit. Since the Earth takes 24 hours to orbit at the equator these satellites stay above the same part of the planet as they orbit. This is called a geostationary orbit, because relative to the surface of the Earth the satellite does not appear to move. These satellites are used for TV stations and communications. They are also used for atmospheric tracking, oceanography and weather observation. These geostationary satellites are at a distance that is almost 6 times the radius of the Earth.
Orbital Period
The closer a satellite is to the surface of the Earth, the less time it takes to orbit. We can see this from low earth orbit satellites only taking 90 minutes to orbit the earth. These orbits are around 400 km high. Orbits at 36,000 km take 24 hours for the satellites to orbit the Earth. It is important to note this principal, that larger orbital paths take longer for satellites to orbit. This can also be seen in the orbital period of the planets as shown in the image below.
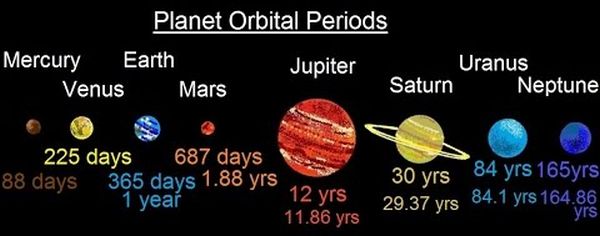
The Challenges of Space Travel
Space is a very different environment to here on Earth. These differences make the exploration of space very difficult and dangerous. Let us look at the physics of why exploring space is so challenging.
Distance
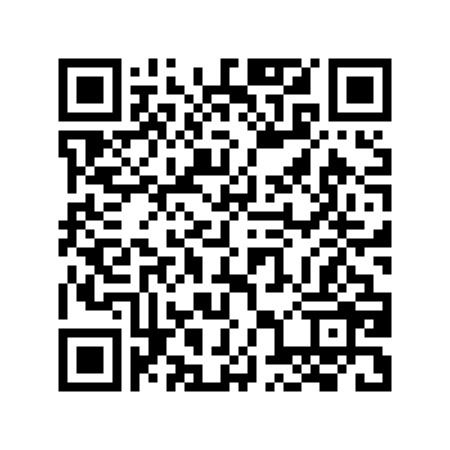
How close is the nearest neighbouring star? What about the closest inhabital planet outside our solar system?
Amongst the fastest objects ever made are the two voyager probes, which have both managed to travel so far that they are outside the influence of our Sun. It took both space probes more than 40 years to travel this distance. At their current speed it would take them over 70,000 years to reach our nearest neighbouring star! In the diagram below are some numbers related to voyager 2 supplied by NASA.
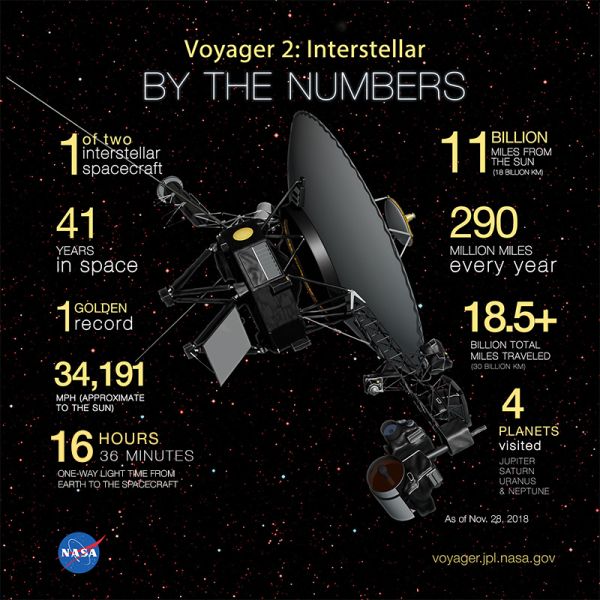
Our closest neighbouring star is proxima centauri at 4.22 light years away. It is a red dwarf star with a planet, "Proxima b" in a habitable zone orbit. Travelling at the speed of light it would take 4.22 years to reach this star. Travelling at the rate of the fastest object we have ever made it would take over 6500 years to reach this star. Astronomical distances are too vast for us to cross with current technology, and so we are limited to the closes neighbouring planets in our solar system.
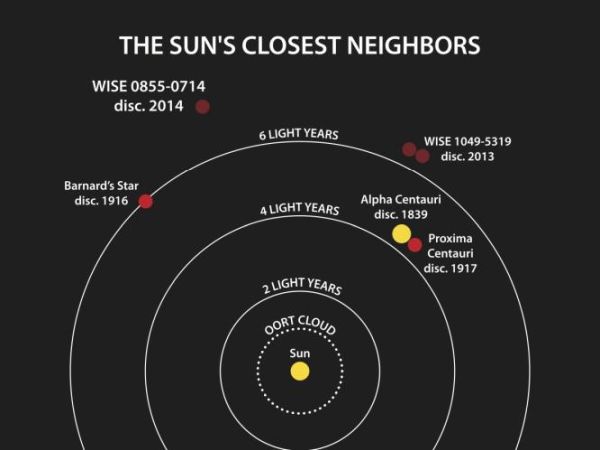
Ion Drive
In order to speed up in space a new type of propulsion system has been invented. It only works in space where there is no air resistance or friction.
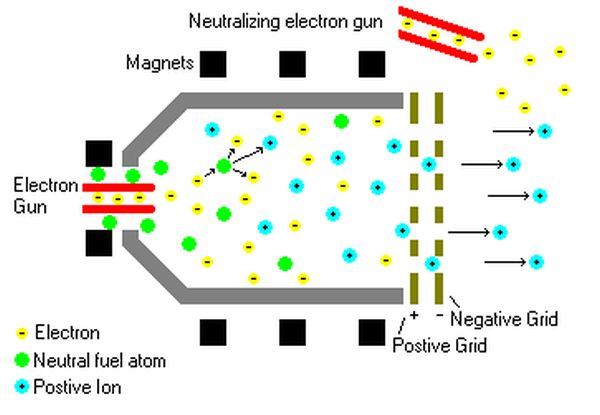
The ion dirve works in following sequence:
- Generate a stream of electrons
- Ionise xenon (an inert noble gas) using the stream of electrons to knock an electron from the outer shell of xenon
- Accelerate xenon ions across an electric field and out of the engine
- Neutralise the ions that have exited to keep the overall system from gaining a static charge
In the third stage they key piece of physics that causes this engine to accelerate is an application of Newton's Third Law of Motion. The third law is that for every action force there is an equal and opposite reaction force. The acceleration of the ions out of the engine causes the ions to accelerate the engine in the opposite direction. The accelearion is very small, but the engine is left switched on for long periods of time, making a very large overall gain in speed.
Gravitational Slingshot
It is possible to speed up space probes like Voyager 1 and 2 by using momentum transfer from a planet, moon or asteroid. This type of manoeuvre is known as a slingshot. As the space probe passes close to a planet the path past the planet is designed so that some of the momentum from the planet is passed to the space probe. Since momentum is given by p = mv, any increase in momentum of the space probe must result in an increased velocity. See the diagram below for the flight path of Voyager 2. The manoeuvre is also called a 'catapult' manoeuvre.
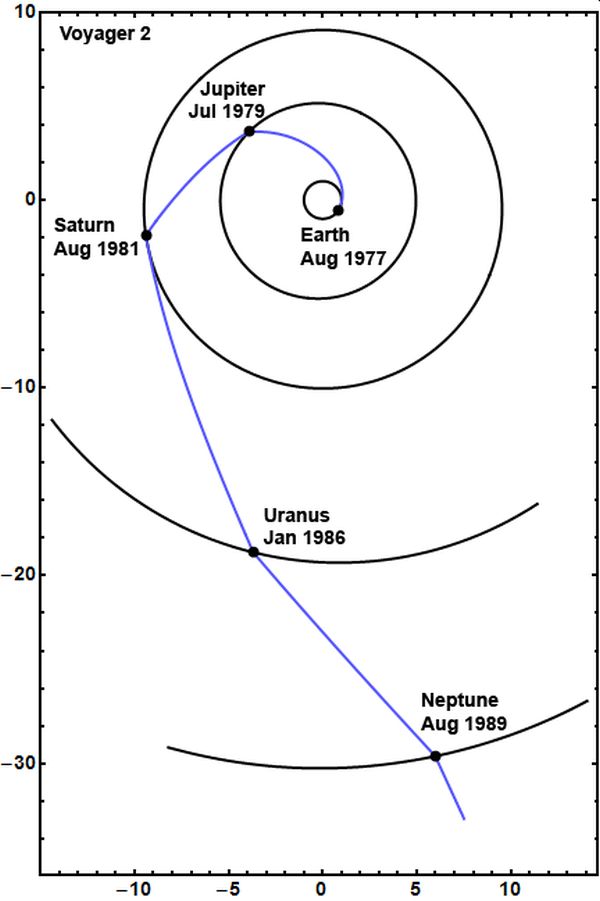
Manoeuvring in Space
If you have ever skated on ice you will know how hard it is to start, stop and change direction. It is more difficult because of the reduction in friction. In space there is no friction, becasue there is nothing to touch. This makes it very difficult to change direction unless jets of gas are used.
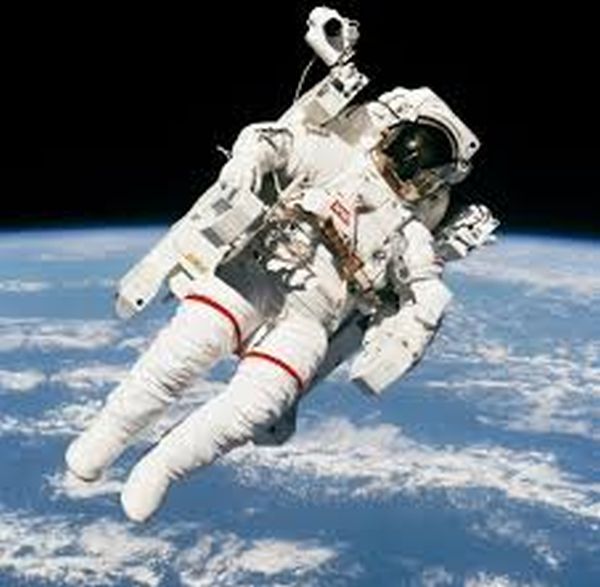
Life support
The fastest objects we have put in space are all unmanned space probes. When it comes to transporting people in space the furthest that they have ever travelled is to the far side of the moon. Keeping the environment habitable is a real challenge, which requires a constant supply of energy. Carbon dioxide cannot be allowed to build up because it is toxic. There must be sufficient heat because out of direct sunlight the temperature of space is just below 3 Kelvin, which is -270° C. One of the key pieces of equipment used in helping to maintain a habitable environment while in space is the solar panel. This panel is capable of converting sunlight directly into electricity, which can be used to power the systems that make up the life support systems. As a space craft gets further from the sun the amount of radiant energy available to the solar panels decreases by the square of the distance. This means that the area of solar panels must be increased greatly for any missions with astronauts to places like Mars, because it is further away from the Sun. The International Space Station is an example of a habitable place in space. It has to be regularly supplied with water, oxygen and food as it is not possible to be self sustaining with current technology.
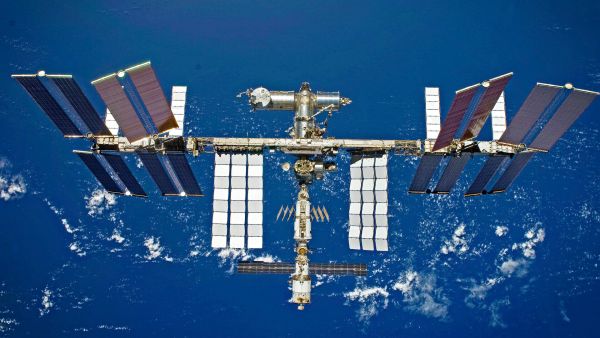
Manned Space Exploration
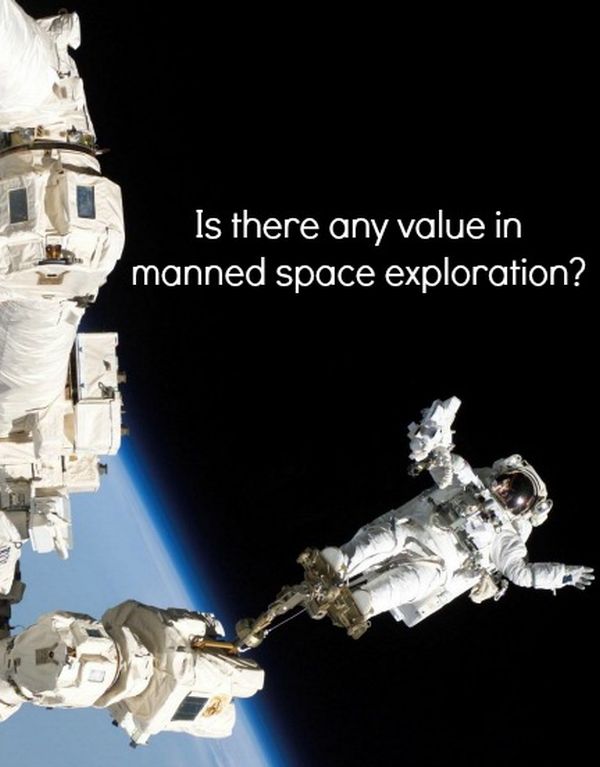
The following aspects will be considered:
- Fuel load on takeoff
When taking off a rocket has a very large amount of fuel and oxidant present. If there are any failures with seals or other aspects of the system the results can be catastrophic. Several astronauts have died due to catastrophic failures at takeoff. Clearly a dangerous aspect in sending astronauts into space.
Falcon 9 rocket blowing up after launch in 2015
Disintegration of Challenger on liftoff 1986 - Potential exposure to radiation
The magnetic field of our planet protects us from harmful ionising radiation present in Solar Winds and Cosmic Rays. When leaving Earth the protection given by the magnetoshpehere is no longer as strong. The further we get away from the planet the greater the effect of harmful radiation on the body. An astronaut taking part in a two year mission to Mars would receive sufficient radiation exposure that NASA would not allow them back into space. - Pressure differential
On the surface of Earth we are so used to the pressure from the air around us that we are generally unaware of the amount of pressure. Atmospheric pressure is 1.01 × 105 Pa. In space the pressure is 1.32 × 10-11 Pa. Upon sudden decompression in vacuum, expansion of air in a person's lungs is likely to cause lung rupture and death unless that air is immediately exhaled. Decompression can also lead to a possibly fatal condition called ebullism, where reduced pressure of the environment lowers the boiling temperature of body fluids and starts changing liquid water in the bloodstream and soft tissues into water vapor. Also consider our dependence on oxygen, which is not present in space. Within 15 seconds, deoxygenated blood begins to be delivered to the brain and you will lose consciousness. By 90 seconds you will be dead. Remember that cooling will take time because there will be no heat transfer by conduction of convection and it will take time for a body to radiate heat energy away.
Our ciculatory system has evolved in a gravitational field. When in space the body experiences microgravity which leads to increased blood flow to the chest and head. Pressure on the eyeball is also increased, leading to a change in its shape. Bone density also requires monitoring because longer periods in microgravity lead to demineralisation of bones.
Marshmallows swell up as the pressure in the bell jar is reduced. - Re-entry through an atmosphere
The capsule that re-enters the Earth's atmosphere is travelling at around 11,000 m/s and must be slowed down. The kinetic energy must be transferred to the atmosphere. It is converted to heat energy through friction between the air and the capsule. In order to reduce the increase in temperature physicists use their knowledge of latent heats to convert kinetic energy into energy that changes the state of a material, rather than leading to an increase in temperature. Silica is a material used because it has a high melting point (above 1600 °C).
Another problem of re-entry is the angle at which the capsule hits the atmosphere. If it is too steep the capsule will descend into the denser layers of the atmosphere too quickly and will incinerate. If it is too shallow the capsule will bounce off the atmosphere, just like a skimming stone bounces off the surface of water. - Energy Sources
Key to being able to survive in space is to have access to energy which will then allow the generation of oxygen and fuel from raw materials. The following types of energy source are commonly referred to in National 5 physics. They are:
Energy Producer Description of use solar panels Useful when relatively close to the sun. Mars is far enough away to reduce efficiency to about half what it is here on Earth. Radioisotope Thermoelectric Generator (RTG) This is a type of nuclear battery which has a long working life. Heat generated in nuclear decay is converted to electricity. 300 to 400 watts of power is available for several decades using an isotope such as 238U nuclear reactors These have been used since the 1960s. They can produce kilowatts of power for times of several months to a few years.
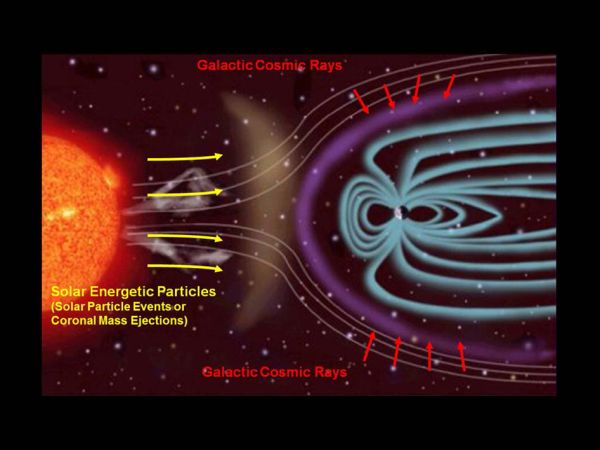
To remind yourself about latent heat and energy transfer you can look at the information on Latent Heat.
Newton's Laws and Space Travel
For a rocket to be able to reach space it has to accelerate from stationary to 11,000 m/s. This means that the thrust force produced by the engines is greater than the weight and air resistance forces. The net upwards force provides the rocket with enough acceleration to increase the speed of the rocket to at leeat 11,000 m/s. This is an example of Newton's 2nd Law of Motion where the acceleration of the rocket is proportional to the net force upwards. The situation is more complex, because for most of the work we do at Nat 5 level, the mass remains constant. This is not so with a rocket, because it burns a lot of fuel and keeps on getting lighter. This improves the acceleration as time increases.
Modern rockets, like the Falcon Heavy, by SpaceX are designed to return to the Earth to be reused. They have to slow down by firing their rocket engine to oppose the gravitational force provided by the gravitational field of the planet which accelerates the rocket to the surface of the planet.
Newton's Third law states that for every action there is an equal and opposite reaction force. This is the principal used in ion drives and rocket engines in space. Ejecting small ions/molecules from the rocket engine results in a reaction force that pushes the rocket in the opposite direction. This is why rockets do not need to push against any medium in space in order to accelerate. They are working on the principal of Newton's 3rd Law, action and reaction.
Example
A rocket of mass 1500 kg fires its engine which produces a thrust force of 25 kN. Ignoring air resistance, how fast does the rocket accelerate when it launches?
Fnet=Fthrust − W
Fnet=25,000 − 1500 × 9.8
Fnet= 10,300 N
F = ma
10,300 = 1,500 × a
a = 6.9 m s−2
Example
A module of mass 850 kg fires its engines which produce a thrust force of 7.5 kN while taking off from the moon. How fast does it accelerate away from the moon?
Fnet=Fthrust − W
Fnet=7,500 − 850 × 1.6
Fnet= 6,140 N
F = ma
6,140 = 850 × a
a = 7.2 m s−2
Note: We do not need to consider air resistance on the moon because it has no atmosphere.
Interactive example 1 and solution
Attempt to solve the question yourself before clicking the solve button!
When the rocket in the interactive example above burns fuel, the total mass being accelerated decreases. This means that the rate of acceleration will increase, because the same amount of thrust force is acting on a smaller mass. This makes calculating flight trajectories for rockets more complicated than has been indicated here.
Satellites in low Earth orbit have a velocity of almost 8000 m s-1. To accelerate to this speed requires very large amounts of energy. This is why we use such large rockets, with so much fuel. It is also the reason that the mass of the satellite is kept as low as possible.